Picture This
Spring 2019
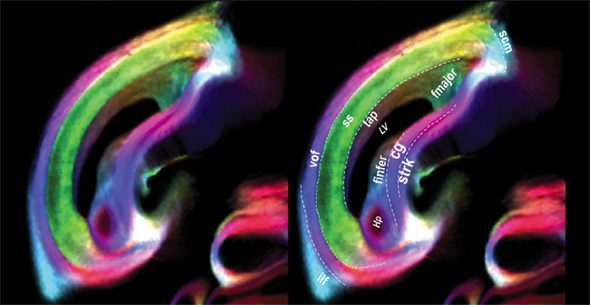
All across your brain, blood vessels are snugly wrapped in a quilt of tightly knit cells known as the blood-brain barrier. But these cells aren’t here to keep your brain warm. Instead, they act like gatekeepers, guarding the brain against intrusion by toxins and other pathogens that would do it harm, all the while granting access to certain other molecules we need, such as oxygen and glucose. The skull gets all the credit for protecting our most precious organ, but we wouldn’t last a day without the blood-brain barrier.
Interestingly, some of the worst neurodegenerative diseases seem to be associated with failures of this structure. This year, 800,000 Americans will suffer a stroke, accounting for a death every four minutes. More than 1 million people in the United States will struggle with some form of multiple sclerosis. In 2018, it was estimated that 5.7 million Americans were living with Alzheimer’s disease.
These are complex diseases, with many molecular actors at play. Yet, Afonso Silva, a neuroscientist and bioengineer who’s spent the past 20 years working at the National Institutes of Health (NIH), also believes: “There might be a common mechanism by which many of these brain diseases work. And one way or another they’re associated with a local inflammation of the blood-brain barrier.”
Silva’s team is offering astonishing new views of compromised brains. He hopes this information will offer some clarity for how to approach neurodegeneration. Despite four decades of research dedicated to studying Alzheimer’s, Parkinson’s, multiple sclerosis, and other neurodegenerative diseases, scientists have yet to come up with a way to do much about any of them.
Part of the problem, says Silva, is that when patients go to the doctor because of symptoms associated with a neurological disorder, the disease is already at a well-developed stage.
At that point, we can diagnose various neurodegenerative diseases. We can track them as they progress. But we are powerless to stop them. And some believe this is because we’ve been looking for clues in all the wrong places.
“The species that is most widely used in biomedical research nowadays is the mouse,” says Silva. “And wonderful things are being learned about the biology of the brain and even about some basic mechanisms of disease.”
Yet, while rodent models have led to innumerable medical insights, many things about them still don’t match up.
For example, to study stroke, many researchers have looked to a human condition known as CADASIL, or cerebral autosomal dominant arteriopathy with subcortical infarcts and leukoencephalopathy. Up to 80 percent of people with CADASIL will experience a stroke by the age of 50. And because the condition is created by the mutation of a single gene, scientists have discovered that it’s relatively easy to produce in rodent models. This should make the disease a home run for stroke research. However, rodents don’t seem to be as affected by CADASIL as humans.
Either the mice don’t stroke spontaneously, says Silva, or the strokes are too small to cause meaningful damage that we can study.
And it’s not just stroke. Scientists have come up with more than 20 different compounds that treat amyotrophic lateral sclerosis, otherwise known as ALS or Lou Gehrig’s disease, in rodents. But none of them have worked in humans.
“A lot of findings in the mouse brain just do not translate to the human brain,” says Silva.
But he has a hunch about some animals that might help us fill that gap. They’re called common marmosets, or Callithrix jacchus—pip-squeak-sized primates with brains remarkably similar to our own.
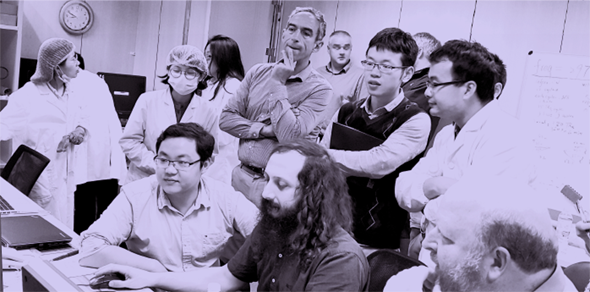
You see, Silva is a virtuoso in the world of magnetic resonance imaging, or MRI. And for the last decade and a half, he and his team at the NIH have been peering into the brains of marmosets at increasingly mesmerizing resolutions. At the same time, the scientists are learning how to tweak the primate’s genome to create models for human conditions. And as of this winter, Silva and seven of his closest colleagues have taken up posts in the Department of Neurobiology at the University of Pittsburgh in an attempt to push this body of work further.
In the next four years, Silva hopes to breed enough working marmoset models of CADASIL to begin to unravel its origins. What’s more, through a partnership with Peter Strick, Distinguished Professor and chair of neurobiology at Pitt, he aims to create the world’s first marmoset model of Alzheimer’s disease. With any luck, the animals will help scientists crack the neurodegenerative disease game wide open.
***
As a kid growing up in Brazil, Silva remembers marmosets as always being nearby. “All you needed to do was look up at the trees, and they would be there,” he says.
In fact, he likens the primates to the squirrels that dominate parks here in the United States. Small, clever, and not above a bit of thievery.
“They are incredibly adaptable animals, so they live really well in civilization. They are experts in identifying food opportunities—and that includes the lunchboxes of unaware students,” says Silva.
To be honest, he never considered that these same rascally creatures might hold secrets in the fight against Alzheimer’s disease, multiple sclerosis, and stroke in humans. Back in those days, practically nobody was looking at marmosets as models for human disease. The animals were thought to be somewhat unintelligent by scientists, says Silva, because they wouldn’t sit for hours on end in a lab and complete repetitive tasks like larger primates, such as macaques. Researchers also preferred macaques because they were seen as being hardier, less susceptible to stress, and capable of more refined hand movements. Of course, rodents were more desired still, because of their small size and cost, rapid reproduction, and ease of manipulation. For many years, Silva’s gaze had settled there, too.
“I had already established a track record of studying the anatomy and function of the rodent brain. But I was getting to a plateau where I wasn’t really learning much more,” he says.
But then, a serendipitous event changed Silva’s course. In 1999 he joined the NIH, where he inherited a colony of marmosets. The researcher who previously had been working with the animals had taken a position in Germany, and the colony was sitting idle.
“I think it was the best thing that happened to me,” says Silva.
While others had shied away from the animals, Silva found them to be surprisingly good study subjects. As adults, marmosets weigh about half as much as a box of dry spaghetti, which makes them easy to handle. They also don’t carry any diseases known to infect humans. In contrast, Silva says, you fear for your life if a macaque sinks its teeth into you, because the animals can carry herpes B, which can lead to meningitis, and simian immunodeficiency virus, which is comparable to HIV/AIDS.
But even more notable is the fact that the brains of marmosets are much more similar to our own than are rodent brains.
“There are many areas of the brain that primates have that rodents don’t. For example, the prefrontal cortex, which [includes more than a dozen] areas that are very important for decision-making and emotions,” says Silva. “These areas are much more developed and more complex in primates than in rodents.
What’s more, the ratio of gray to white matter found in the marmoset is also very similar to that of a human. This is important, because what we refer to as “white matter” is actually the long, reaching arms of the neurons, called axons. Brain cells use axons to trade electrical impulses with one another, and in some cases, axons can be found snaking their way across entire sections of the brain, allowing for one part of the organ to communicate with another. But to conduct electricity across such wide expanses, axons have to insulate themselves with a white layer of fat called myelin.
Rodent brains don’t have much white matter though, because they’re so small. This is fine for the mice and rats, of course, but it makes the animals less than ideal for studying human diseases that manifest in white matter, like multiple sclerosis.
The other interesting thing about marmoset brains is that they lack the wrinkles most people picture when thinking of a human brain. But this difference in appearance is actually a boon, because wrinkles can get in the way of good imaging.
“The main advantage of having a smooth brain is that I can easily locate on the surface of their brain any functional area that I want. So if I want to study the somatosensory system, the sensation of touch, it’s right there; or the visual cortex, it’s right there. The prefrontal areas also are very easy to access just because I don’t need to deal with the convolution of the cortex,” says Silva.
The proof is in the pudding. Throughout the past several years, Silva and his colleagues have pushed MRI technology to new limits by creating images of marmoset brains at resolutions down to 80 micrometers isotropic, which means that the images created at this resolution can be three-dimensional.
To put this into perspective, that’s a resolution about 60 times better than what is possible in macaques, says CiRong Liu, one of the neuroscientists Silva is bringing with him from the NIH. And it’s 4,000 times better than what’s being used in the Human Connectome Project, which is a collaborative effort to map the human brain at high resolution.
Put another way, not only can the team see nerve fibers, but they can also see the direction in which the fibers are arranged. It’s sort of like looking at a map of the United States and being able to zoom in and note the flow of traffic on a highway.
“In the white matter, we’re seeing structures we were never able to see previously in human data or macaque data,” says Liu.
In 2017, Liu and Silva published their work in the journal NeuroImage, providing a high-resolution, three-dimensional atlas of the marmoset brain. Such an atlas had not existed before. In all, they mapped 54 cortical areas and 16 subcortical regions, which gives them and other research teams a road map that can be used to pinpoint areas of importance. Even to a layman’s eyes, the atlas is gorgeous—like looking at the neon greens, pinks, and yellows of a soap bubble. And the crispness with which you can make out structures compared to similar images taken in macaques and humans is plain to see.
In part, images like these are only possible because marmosets are extremely small, as primates go. Not including its skull, a marmoset brain is only about as big as a shelled pecan, and it weighs less than a AAA battery. Even still, it took one of the most powerful MRI machines on the market nearly one week to record brain structures in that kind of detail.
But the marmoset brain atlas becomes even more useful when you add in the work of JungEun Park. “She’s the one who has eyes in her fingertips,” says Silva.
***
Unlike Silva and Liu, who specialize in imaging, Park is an expert in genetic engineering. Using a variety of techniques, she can add to, delete from, or otherwise annotate an animal’s genome to make it more relevant for study.
It’s a slow, painstaking process laden with disappointment. After Park performs her manipulations, she surgically implants the transgenic embryos back into a surrogate mother. Then, she must wait for nature to take its course, which in common marmosets is about five months. Unfortunately, the odds of survival are not good.
“Marmosets naturally have a high percentage of early stage miscarries. We don’t know why,” says Park, who will join Silva and Liu at Pitt this summer.
Out of every 10 transfers, just two to three will result in pregnancies. Then the team must wait another 18 months for the animals to become mature so that the marmosets can participate in studies or contribute to the next generation of transgenic models. It’s a long time compared to mice, which take only around two months to reproduce and reach sexual maturity. But as far as primates go, marmoset reproduction is about as quick as it gets. Depending on the species, macaques require three to seven years from conception to sexual maturity, for instance.
The good news, says Silva, is that female marmosets can produce two litters each year and most often give birth to twins. Triplets even happen on occasion. All of which makes marmosets sort of like the rodents of the primate world.
At the NIH, Park, Silva, and colleagues used these techniques to not only create a marmoset model of multiple sclerosis, but also to track that animal with MRI scanning as it developed a lesion in its white matter.
“You don’t know where to look before the lesion is there,” says Silva, “but once the lesion forms, and you have those coordinates, you can go back in time and say, ‘Aha!’”
In fact, in a study published in 2014, the scientists were able to use this retrospective approach to find evidence that the blood-brain barrier had been compromised two to three weeks before there was any other visual evidence of the lesion in the scans.
“It was allowing things that should not cross the blood vessels to cross the blood vessels,” Silva explains.
All of which leads the team to hypothesize that if scientists could develop a technique that’s super sensitive to permeability of the blood-brain barrier, they might be able to predict where and when a lesion will appear. Then doctors might use steroids or other drugs to slow it down, and with it, the progression of multiple sclerosis.
In a similar fashion, Silva is confident his team will be able to study the development of Alzheimer’s disease in marmosets. Despite being small and reproducing relatively quickly, the animals can live well beyond 10 years of age. There’s also evidence that later in life they start to develop amyloid plaques and neurofibrillary tangles—irregularities that are hallmarks of Alzheimer’s in humans. What we don’t have evidence of just yet is that these lesions affect the marmosets in any meaningful way. However, researchers might be able to accelerate this aging process by engineering a transgenic line of marmosets that expresses mutant presenilin, a protein scientists have connected to early onset Alzheimer’s disease.
As exciting and promising as marmoset models are, it’s important to note they are not perfect.
For instance, just as presenilin is linked to early onset Alzheimer’s, a protein known as A53T alpha-synuclein has become associated with the rise of early onset Parkinson’s disease. You might think that by adding such a protein to the marmoset genome you could reliably create a model in which to study Parkinson’s.
But this isn’t the case, says Marina Emborg, director of the Preclinical Parkinson’s Research Program at the Wisconsin National Primate Research Center, because marmosets already express this protein naturally.
“It’s not a mutation for them, and they don’t have Parkinson’s,” Emborg explained at a meeting of the National Academies of Sciences, Engineering, and Medicine in October.
All models are wrong, goes the oft-repeated aphorism in statistics, but some are useful.
***
“When Dr. Silva first started combining MRI and molecular genetics in the marmoset, it was a bit of a gamble because very few people in the world worked in either MRI or genetics of the marmoset,” says Alan Koretsky, chief of the Laboratory of Functional and Molecular Imaging at the National Institute of Neurological Disorders and Stroke and Silva’s former boss. But it was a risk that’s paid off. Koretsky notes that many groups are moving ahead with these approaches.
“I think progress in biomedical research really has relied on asking the right question, in the right model, at the right time, and with the right tools,” says Koretsky.
Given where things now stand with advances in imaging and genetic analysis, Koretsky believes Silva and others in the field are on the cusp of breakthroughs in understanding neurodegenerative disease. “I think there’s a sense everywhere that we have been making rapid progress,” he says, “and that progress is going to translate to an impact sooner or later.”
It may be that no one is more eager about the addition of Silva’s team than Arthur S. Levine, Pitt’s senior vice chancellor for the health sciences and Petersen Dean of the School of Medicine. He will be opening a lab that studies Alzheimer’s. Once a successor is found to fill Levine’s sizable shoes, he plans to step down from his administrative responsibilities, after 20 years, and work alongside Silva and Strick in the search for clues to neurodegeneration. (More about Levine’s new career as a neuroscientist in a future issue.)
Peter Strick is also more than delighted to have Silva on board. “Afonso has got incredible imaging skills,” says Strick. “What he’s able to do in terms of resolving the brain structure is just remarkable.”
Now, Strick wants to use Silva’s skill set to complement his own work mapping unexpected neural connections throughout the body, such as those between cells in the retina and regions of the brain associated with depression and others in the liver involved with metabolism.
He compares these kinds of collaborations to the parable of the blind men and the elephant. “You can look at things from different perspectives, and it’s when you combine those perspectives that you get powerful insight,” says Strick. “[Silva] started [in December], and we had a grant in on January 25. I can’t wait. He’s already changed some of the direction of my research, and I’ve already changed some of the direction of his.”
For Silva, who completed his PhD in biomedical engineering at Carnegie Mellon University in 1996, the return to Pittsburgh feels like his whole career is coming full circle.
“It started here. It will likely end here.”
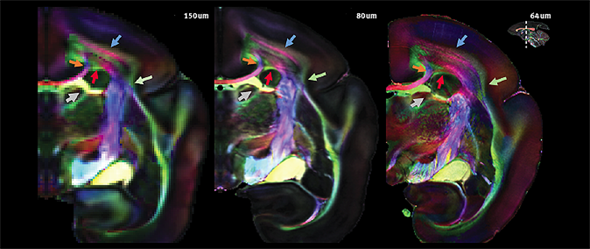
Brain images courtesy Cirong Liu, John Newman, Frank Ye, Cecil Yen, Daniel Glen, Diego Szczupak, Piotr Majka, Joseph Guy, Seung Kwon Ha, Marcello Rosa, David Leopold, and Afonso Silva.