How symmetry arises
Winter 2018

In a chaotic world, we humans crave order. We seek out patterns, then contemplate their deeper meaning. Symmetry—like the bilateral mirror imaging that characterizes our facial features, our brain hemispheres, our limbs—is perhaps the most basic of the patterns found in the natural world, contributing to both our survival and a wealth of discovery.
That creature partially obscured behind a tree trunk? We can fill in the blanks to deduce its size, the breadth of its horns, or the number of claws it may bare if we get too close. That mountain rising up in our path? We assume that if we can make our way to the top, there will be a route back down.
Greek philosophers pronounced such balance a core feature of aesthetic perfection. “The chief forms of beauty,” wrote Aristotle in Metaphysics, “are order and symmetry and definiteness.”
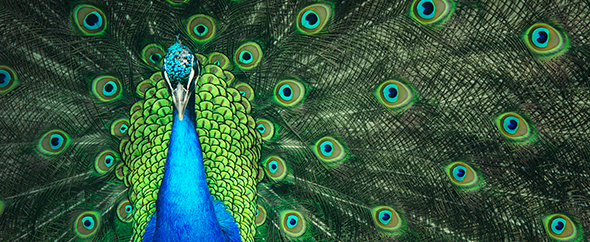
The iridescent train of the male peacock exemplifies Aristotle’s ideal. And yet its beauty utterly confounded naturalist Charles Darwin. According to prevailing philosophies of his time, such splendor was a gift from God, a foretaste of heavenly glory here on Earth.
But in a world ordered instead by natural selection, what was the point of such a gaudy display as the male’s extravagant plumage?
“The sight of a feather in a peacock’s tail, whenever I gaze at it, makes me sick,” Darwin lamented in an 1860 letter.
It would take the naturalist another decade to dial in on the evolutionary function of such seemingly frivolous elaborations: sexual selection. Such displays, he proposed, broadcast an individual’s fitness, boosting one’s chances against reproductive rivals. Bighorn ewes pick rams whose racks live up to the species’ moniker; even the honeybee has a penchant for balanced blooms.
This isn’t just about us critters, by the way. Pythagoras hypothesized that the planets must be spherical, the most symmetrical shape of them all. Indeed, symmetry prevails in the physical structures of the natural world—reflected in Einstein’s theory of relativity and visible to the naked eye in the webs spun by orb spiders, the patterns frozen within each snowflake, the nests built by barn swallows. Start looking, and you’ll notice it everywhere.
Okay. So, what about us? What of the animal kingdom? Does the symmetry of our body plans convey some fundamental reproductive advantage? Is it a byproduct of something deeper—say, genetic architecture, or even an echo of the laws of physics that govern the material world? Deep inside our every cell we harbor the same elements that make up the vast universe.
It’s fun to ponder such questions, as Xiangyun Wei has done for a while. He offers this: It appears that, for vertebrates, the coming and going of symmetry propels healthy development.
Back in the mid-’00s, Wei was digging into the process by which a sheet of epithelial cells within a zebra fish embryo differentiates, giving rise to the retina. In humans and zebra fish alike, that process begets a pair of light-sensitive retinas that initiate the gift of vision. In some cases, however, the process unravels, and the resulting disorder within the retina causes congenital blindness.
An associate professor of ophthalmology at the University of Pittsburgh with joint appointments in developmental biology and in microbiology and molecular genetics, Wei wanted to understand the progression of molecular events involved. Very early in vertebral development, the fast-dividing cells of an embryo give rise to primordia of many organs. Among them is the neural tube, a precursor to the central nervous system, including the brain, the spinal cord, and the retina. During embryogenesis, a brisk process of cell proliferation, reorganization, and differentiation introduces the asymmetry that yields heads and tails—while maintaining symmetry across the left and right halves of the body. And somehow fully formed, functional retinas end up right where they belong. Wei studied the genes that transcribe certain proteins (like N-cadherin and Lin7c) implicated in pushing and pulling the optic system into existence. He found that the embryos with certain genetic manipulations were likely to die well before their retinas could fully differentiate. Necropsy revealed a fatal flaw. Says Wei: “The mirror symmetry of the neural tube was messed up.”
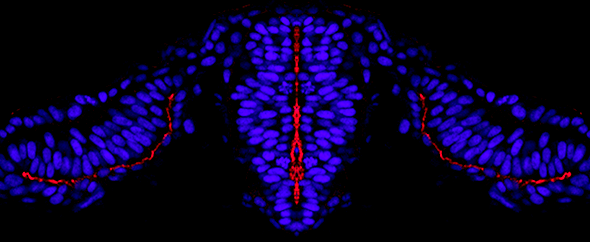
For example, an early and heavy-handed manipulation of Lin7c resulted in a chaos of branches akin to the six-pointed stars that feature in the old-time children’s game of jacks—instead of an orderly, functional nervous-system-to-be. This suggested the importance of expressing polarity proteins at specific points in time. He and former postdoc Xiaojun Yang published these results in 2009.
Among the dead embryos in Wei’s lab, clearly something had gone very wrong. And, for Wei, these shenanigans pointed to a paradox of metaphysical import: How does symmetry ever emerge in a biological system that embodies mobile, asymmetric components?
Says the scientist: “That’s bugged me for a long time.”
Nearly a decade, in fact. In May 2018, iScience published a follow-up by Wei and his team (including first author postdoc Chuanyu Guo) revealing key elements that form the foundations of bilateral symmetry of the zebra fish central nervous system.
Other investigators had hypothesized that the mere fact of cross-midline cell division during neural tube formation led to bilateral symmetry. (Each pair of daughter cells splits across a midline.) But that theory didn’t account for the intricate choreography across time and space of myriad proteins known to reposition cells within the embryo.
In their iScience paper, Wei’s team homes in on the role of “apical adhesions,” the bonds that form and dissolve as they coax asymmetric cells into positions of polarity and oppositional alignment. The process creates mirror symmetry, and the mode of the adhesions at work seems to be relevant to the development of many vertebrate tissues.
Wei offers a unifying theory to explain how tissues within the growing embryo simultaneously maintain integrity and also allow the plasticity necessary for fast-dividing cells to change shape and position.
“According to Taoism,” says Wei, “everything is made of two things—a yin and a yang, a positive and a negative. They have to interact with each other and sometimes they interchange from one property to another.” Only through reciprocal interaction does an entity achieve unity—whether the entity is the neural tube, the gut, or even, says Wei, the two-party system at the heart of American democracy.
You always want to have two things interacting with each other to allow development, Wei adds. “Many tissues and organs start with either a tube or a sac. [That] naturally creates two opponents. These two opponents interact and direct each other to develop.” In the zebra fish and in humans, he says, “the RPE [pigmented cell layer that nourishes the retina] and retina start from the optic vesicle, like a ball, or a balloon. At a certain point, the balloon collapses and forms a cup, at which point there’s an inner and outer layer. The outer layer becomes the RPE. The inner layer becomes the retina. Starting from the optic vesicle, it looks like just one thing; and by changing the folding pattern, you generate two opposing components.” You get differentiation.
“They started as something very similar, and become two things very different,” says Wei. “And the two tissues still interact with each other.
“Nature is very smart.”
Where symmetry breaks
Symmetry may surround us, but mathematicians—Alan Turing most famously—become fascinated at the points where it breaks down.
Pitt’s Bard Ermentrout wrote the book Mathematical Foundations of Neuroscience. A University Professor of Computational Biology, he is interested in patterns and the universal mathematics that undergird slips in symmetry. “Left- and right-handedness are a kind of breaking of symmetry,” he says. “If we were completely symmetrical, we would look like really ancient tubeworms.”
The cascade of events that take place in embryogenesis has long been imagined as a process of pure symmetry breaking, as a patterned body plan emerges from a single cell, notes Ermentrout. In their iScience paper, Pitt’s Xiangyun Wei and Chuanyu Guo detail how mirror symmetry emerges in neural tube formation. (See story above.) At another point in development, Ermentrout points out, “zebra fish [embryos] form somites, a series of segments. You’re breaking the symmetry by adding more things you have to keep track of.”
And yet pure asymmetry is vanishingly rare in the natural world, says Ermentrout. —SRT
--
Photography by Getty Images.
Zebra fish image courtesy Wei Lab.