The travails before the trials of a promising treatment
Spring 2021

Before John Mellors and Dimiter Dimitrov, founding director of Pitt’s Center for Antibody Therapeutics, launched their UPMC-backed Abound Bio, they surveyed the clinical and business landscape. “I initially thought we would target infectious diseases,” says Mellors, who is chief of Pitt’s Division of Infectious Diseases and director of UPMC’s HIV/AIDS Program. “But Dr. Dimitrov and I decided there was greater unmet medical need in cancer.”
This was, perhaps obviously, in the era b.c.—before COVID-19. October 2019, to be exact.
In January 2020, Dimitrov, a University of Pittsburgh professor of medicine, and his lab were in the midst of several pediatric cancer projects. His group had also advanced to clinical trials an antibody that neutralizes Hendra and Nipah infections (respiratory viruses circulating in Australian fruit bats that have crossed into humans).
Suddenly his circles were abuzz with news of a pneumonia outbreak in China. “My collaborators are at the Wuhan Institute of Virology, where the virus was sequenced,” he says.
When Chinese scientists released the genome of SARS-CoV-2 in mid-January, Dimitrov told Mellors he wanted to redirect his research team to the novel coronavirus.
They could find an antibody to stop it in record time—if his boss was willing to sign off.
This wasn’t Dimitrov’s first tango with a coronavirus. In 2003 he had zeroed in on how SARS-CoV infects people, then found an antibody to block it. He’d done the same with MERS-CoV. Now his team at Pitt was ready to tackle COVID-19.
“Mitko’s great,” says Mellors of Dimitrov. “He always asks me what I think.” Their conversation about the novel coronavirus, however, was short. This is an emergency, said Mellors. Go right ahead. Full force. UPMC Enterprises pitched in to cover the costs.
Why was Dimitrov—and colleagues who knew of his team’s work—so convinced he could stop the novel coronavirus?
Mellors recruited Dimitrov from the National Cancer Institute (NCI) in 2017. The time was ripe to exploit advances in antibody engineering to give the immune system the upper hand against a wide range of chronic maladies like HIV, cancer and rheumatoid arthritis. In his 27 years at NCI, Dimitrov had developed and refined methods to build vast libraries of engineered human antibodies, then identify the best among them to tweak immune function as needed for a condition.
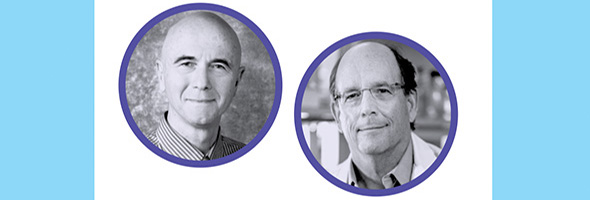
A little immunology background: The adaptive immune system learns by exposure to antigens, the surface molecules that distinguish friend from foe—a beneficial gut bacterium gets a pass; E. coli gets the boot.
Viruses, bacteria and the like mutate fast, and the adaptive immune system has to be ready for anything. So our blood churns out a diverse array of antibodies. Dimitrov focuses on the most common, a class of y-shaped proteins known as immunoglobulin G (IgG). Each form (or “isotype”) of IgG has a unique binding site at its tips.
When one of these binds to an antigen (like a lock and key), the combination serves as a homing beacon for the immune system’s search-and-destroy department.
Not every key fits every lock with equal precision. Antibodies vary, too, in the rate and strength of their binding action.
The human body takes a more-is-more approach, with a weighty ring of keys on hand for whatever may come. Some antibodies may bind only partially to a given antigen, others not at all, but with enough forms in the mix, they collectively get the job done. The goal for therapeutic antibody applications, by contrast, is to find a single form—a monoclonal antibody—uniquely suited to the task at hand.
If you’re going to count on just one protein to derail a fast-mutating virus like HIV—or SARS-CoV-2—you need one strong enough to bind to its target, but not so specific that a new variant will escape its grasp, explains Wei Li, a Pitt assistant professor of medicine and assistant director of the Center for Antibody Therapeutics.
Dimitrov and Li start with what amounts to a replica of the adaptive immune system in their laboratories. They turn human B cells into tiny factories, cranking out trillions of IgG antibodies, each with a distinctive binding pattern.
The resulting library approximates a vast universe of antibody possibility, explains Li.
Known as “naïve,” some of these antibodies may have existed in the original patients, but many of them are new because of the random pairing of the two major components of the antibody: its heavy and light chains. Yet they’re made from fully human components, so there’s no risk of a future patient having a cross-species reaction. (Early recombinant antibodies contained mouse proteins that triggered allergic reactions in humans.)
Because some of the antibody components generated by human DNA tend to aggregate quickly in the lab environment, Dimitrov and Li do a good bit of behind-the-scenes biochemical engineering. While some investigators prefer mouse proteins because they’re less finicky, the Center for Antibody Therapeutics team has developed scaffolds and other tools to stabilize the gene products in their libraries.
For his team, finding the right monoclonal antibody for a given job is akin to, as Dimitrov likes to say, panning for gold. They apply a technique known as phage display, which garnered the 2018 Nobel Prize in Chemistry for George P. Smith and Sir Gregory P. Winter.His team modifies a bacteriophage (which is, oddly, another virus) to express a particular antibody on its surface.
Then the researchers make a library of hundreds of billions of antibodies expressed on the surfaces of different phage particles. They mix the library with the antigen, in this case part of the SARS-CoV-2 spike protein. Antibodies that bind to the antigen become like a weight. “So we can pull down the particles that are heavier,” says Dimitrov. “That’s how we separate them from the other billions of not-important antibodies for a particular purpose.”
The first round of panning might yield hundreds, or even thousands, of antibodies of interest. Subsequent rounds narrow the field. Says Dimitrov: “Typically, after three or four rounds of panning, which takes about a week, we identify the strongest binders.”
In their quest to thwart SARS-CoV-2 infection, the Center for Antibody Therapeutics had one additional advantage beyond the array of libraries, search strategies and tools already on hand. In 2003, SARS was spreading around the world. Dimitrov managed to characterize the molecules on its spike protein that the virus uses to infect a human cell, by way of the human ACE-2 receptor. The SARS-CoV-2 genome revealed that it was using a very similar approach to initiate COVID-19.
While he was still at the NCI, Dimitrov had built a phage to express the SARS glycoprotein and identified an antibody that stopped it from binding to the ACE-2 receptor, like capping a hypodermic needle to prevent an accidental stick.
Given the similarity between SARS and SARS-CoV-2, additional modifications to the tools were fairly straightforward. By early February 2020, the team at Pitt had two candidates—dubbed Ab1 and Ab8.
Soon after, they showed that each was effective against the novel coronavirus in a petri dish.
Publication of the requisite findings in animal models, however, would take months. Wild-type mice aren’t susceptible to infection by SARS—or SARS-CoV-2—because they lack the ACE-2 receptor. Humanized mice and hamsters had been widely available in 2007 when Dimitrov tested his SARS antibody, but by early 2020, few remained. In early 2020, research facilities scrambled to breed their remaining animals, then waited months for them to mature.
The peer-reviewed papers on Ab1 and Ab8 appeared in Cell, PNAS and Vaccine in the fall of 2020. The technology to produce Ab1 and Ab8 had already been patented, so Pitt promptly licensed it to Abound Bio, raising much-needed funds to fuel future research. Dimitrov and Mellors hope one day the breakthroughs will help patients. The journey from drug discovery to U.S. Food and Drug Administration (FDA)–approved treatment can be arduous, however, and licensing is only the first step.
As the world marked the one-year anniversary of the pandemic’s start, Dimitrov was eagerly awaiting data on the feasibility of pharmaceutical-grade manufacturing for Ab1 and Ab8, the first step toward clinical trials. While the FDA had granted emergency use authorization to Eli Lilly and Regeneron for their antibody cocktails, Dimitrov held out hope for Ab1 or Ab8 to demonstrate comparative advantages in their formulation, efficacy or delivery.
(Ab8, for example, is so small it might be delivered by inhalation. By contrast, the currently authorized treatments require an hour-long intravenous infusion.)
Dimitrov believes that the wait for appropriate animal models to verify Ab1 and Ab8 cost lives as the pandemic spun out of control in the United States.
“I was very emotional. I continue to be emotional. We were the first to have antibodies because our method is the fastest, but we will be the last to put them in humans.”
Although the monoclonal antibodies Dimitrov identified to stop SARS and MERS were licensed years ago, neither has been formulated for clinical trials. The business case for development dried up when case counts fell. Arguably, however, the public health case persists. Pandemics don’t send hold-the-date notices.
Dimitrov champions the cause every few years—most recently, in 2017 when he coauthored a call to action in Frontiers of Medicine with collaborators in China. “Outbreaks of emerging infectious diseases pose serious challenges to global public health,” they wrote.
In their view, monoclonal antibodies are a viable tool for halting outbreaks, protecting front-line workers and saving lives. Antibodies have both prophylactic and treatment potential. To realize their potential, however, governmental, academic and industry players would have to collaborate to refine technologies and subsidize costs.
The Center for Antibody Therapeutics has largely resumed its cancer-related monoclonal antibody projects with some exciting prospects—including molecular targets in liver cancer and ways to improve existing cancer immunotherapies. With researchers at Tsinghua University, they figured out how to control tumors and prevent “T-cell exhaustion” so the body can better fight cancer. Abound Bio and China’s Yufan Biotechnologies are moving toward clinical testing.
As a boy in communist Bulgaria, Dimitrov devoured the biographies of pioneering immunologists Louis Pasteur, Robert Koch and Paul Ehrlich, the German originator of the “magic bullet” theory that undergirds contemporary targeted therapeutics.
“Since 8 years old,” says Dimitrov, “my dream was to become a scientist and save people, like they had.”